Toxicology 101: The Basics
Table of Contents
1. What is toxicology?
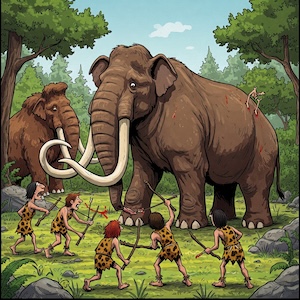
Toxicology is the study of the injurious / adverse effects of substances on biological systems. It involves investigating adverse effects of chemical, physical or biological agents on living organisms and the ecosystem. This includes assessment of approaches to prevent and ameliorate the adverse effects of these substances.
As a discipline, toxicology is a multidisciplinary integrative science that seeks to incorporate information from multiple fields (biology, physiology, chemistry, etc.) to help preserve and protect health and the environment from the hazards presented by chemical and physical agents.
Historically, man has known about the poisonous effects of plants and venoms since primitive times, with this knowledge ranging from pre-historical hunter-gatherers to the modern pharmaceutical revolution. Indeed, the term toxicology descends from toxicon, which refers to poisonous substances into which arrows were dipped. Even to this day, arrow poisons like curare are used as paralytic agents by hunter-gatherer tribes in the Amazon. Moreover, knowledge of the adverse effects of arsenic and opium have been recorded in ancient India since approximately 900 BC.
It was the 16th century Swiss physician and philosopher Paracelsus who elaborated the relationship between poisons and medicine with the saying that all things are poison, and nothing is without poison, the dosage alone makes it so a thing is not a poison
. Toxicology came to be considered as a distinct field through the work of the 18th century Spanish physician Mathieu Orfilia (considered the father of modern toxicology) whose work (notably his treatise on poisons) focused on forensic toxicology. Later, Claude Bernard hypothesized that knowledge of physiology could be enchanced by understanding how toxicants affected biology.
2. Some basic terms and definitions
- Poison / toxicant: Any chemical / substance / test material that can exert an adverse outcome on a biological system
- Toxicity: Inherent ability of a toxicant to exert its adverse effects (often assessed via defined in chemico, in vitro or in vivo assays)
- Toxin: This term can be synonymous with "poison" or "toxicant", but is frequently used to refer to those toxicants produced by biological systems
- Venom: A mixture of toxins produced by an organism (e.g., those produced by certain species of snakes (like those of the genus Naja (cobras)), wasps, spiders, etc.)
- Xenobiotic: Derived from the Greek word "xenos" (stranger). The term xenobiotic means "stranger to life" and denotes a foreign substance administered to an organism or biological system
- Dose: The amount of a test material that is administered to a biological system at a defined period or point in time. Dosage depends, together with other factors, on the concentration of the toxicant as well as the length, frequency and route of administration. Freuqently, doses are normalized relative to body weight and expressed in units of mg of test material per kg body weight (mg/kg bw)
- Dosage: Dose administered per unit of time (e.g., mg/kg bw/day)
3. Some basic concepts in toxicology
3.1 The dose-response relationship
A foundational principle in toxicology is the concept that "the dose makes the poison". As such, the intensity of toxicant depends on its concentration at the site of action, which in turn depends on the dose to which the animal or system has been exposed.
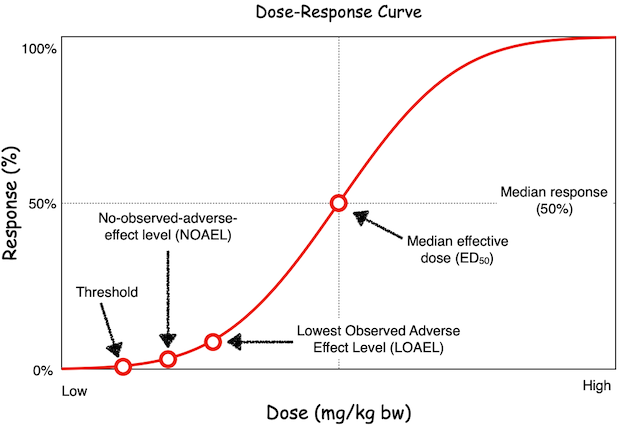
The fact that all substances are toxic at a sufficiently high dose implies that even apparently innocuous substances such as water can be acutely toxic at high levels of exposure. Indeed, the median acute lethal dose (termed the LD50) for water via the oral route is over 5 L for a 60 Kg adult human [2]. On the other hand, certain compounds like potassium cyanide are highly acutely toxic, with an oral LD50 value of around 5 mg/kg body weight (approximately 300 mg for a 60 kg adult) [3], which is over four orders of magnitude more potent than water.
When analyzing median lethality data (or indeed, any toxicological dose-response data), one concept to keep in mind is that the lower the value the more potent the substance. For example, potassium cyanide, with an acute oral LD50 value of around 5 mg/kg bw is over 1000-times more acutely toxic than ethanol (grain alcohol) whose acute oral LD50 value is over 7,000 mg/kg [4].
Typical dose-response relationships are assessed as cumulative proportion of adverse effects as a function of dose dose. Figure 1 shows a typical (idealized) dose-response relationship. As can be seen, this is a sigmoidal curve, which corresponds to the cumulative response seen over a normal distribution (bell-curve) of tested doses. In most cases, the dose-response curve may present a threshold, which is a minimum dose that is required before a measurable effect is observed. From a dose-response curve, several additional toxicological dose descriptors can be calculated:
- The median effective dose (e.g., a median lethal dose (LD50), median effective dose (ED50), etc.,): The median dose producing the measured effect. In the case of measuring lethality, the measured value is termed the LD50. Median effective doses are usually reported in units of mg of toxicant per kg bw (especially for oral dosing).
- No observed adverse effect level (NOAEL): Highest tested dose at which no biologically (or statistically)-relevant adverse effects are visible. NOAELs are typically reported in units of mg of toxicant per kg bw per day.
- Lowest observed adverse effect level (LOAEL): Lowest tested dose at which biologically (or statistically)-relevant adverse outcomes are visible. LOAELs are typically reported in units of mg of toxicant per kg bw per day.
The NOAEL and LOAEL values from the dose-response relationship obtained from a repeat-dose study (e.g., a 28-day study) can be used to determine a point of departure (POD), which represents the first dose at which a biological response is observed. The POD is used to derive health-based guidance values such as the acceptable daily intake (ADI), which takes into account the dose descriptor (e.g., the NOAEL), along with uncertainty factors (UFs) and, if deemed necessary, other modifying factors (MFs). UFs are typically included to account for inter- and intra-species differences and allow for extrapolating from animal studies to the human population. Additional UFs may be added as deemed necessary, such as when extrapolating from sub-chronic to chronic NOAELs, etc. A MF is an additional uncertainty factor that is included to account for other uncertainties, taking into account professional judgement from subject matter experts based on mechanistic, pharmacokinetic or other data.
In certain rare instances, a non-monotonic dose-response curve may be observed. Such a relationships appears like an inverted bell-curve with effects seen at very low and very high doses, representing distinct responses at different dose ranges. In most cases, such effects are due to multiple underlying mechanisms and the should be investigated in more detail by trained subject matter experts.
3.2 Understanding hazard and risk
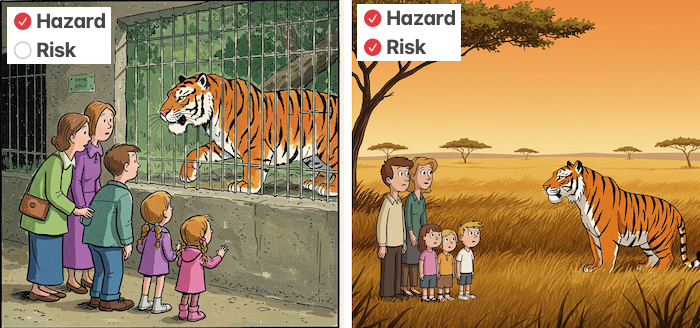
A hazard is defined as probability or practical certainty that adversity would occur when an organism or biological system is exposed to a xenobiotic / toxicant at a defined quantity and under a defined set of conditions. As such, a hazard can be considered to be the potential for a chemical or xenobiotic to cause harm.
On the other hand, risk can be defined as the likehood for a hazard to exert its adverse effects on a biological system or organism. Assessment of risk needs to take into account the presence of a hazard as well as the potential for a meaningful exposure. Thus, a hazardous substance with zero exposure potential will likely pose a very low (or indeed, negligible) risk. In addition to the exposure potential, the toxicokinetics of the test substance needs to be considered when undertaking a risk assessment since xenobiotics that are unable to be absorbed or those that are rapidly eliminated may be indicative of a lower risk potential (one exception to this would be xenobiotics that are metabolically activated to the active form (e.g., tri-ortho cresyl phoshate (TOCP), which needs to metabolically activated to the active cyclic saligenin phosphate form in order to act as an inhibitor of neuropathy target esterase (NTE))).
As illustrated in Figure 2, one way to think about the concepts of hazard and risk is to consider an encounter with a tiger: If this occurs at the zoo, the presence of the tiger presents the possibiity of harm to an observer (i.e., a hazard). However, an observer outside the animal enclosure has a negligible risk since there is a barrier between him and the tiger, thereby shielding the observer from attack. On the other hand, if the observer were to encounter the tiger in an open field, this represents both a hazard (from the presence of the tiger) and a risk (since the tiger is in an environment lacking any physical barriers and is therefore able to attack the observer). Similarly, many innocuous substances (such as pure water) can be hazardous (e.g., being able to cause acute lethality) at sufficiently high doses (in the case of water, the LD50 is estimated to be over 5 litres for a 60 kg adult human). However, at the levels at which we normally consume water (approximately 2 litres per adult human per day), the risk of acute lethality from consuming water is minimal (note: this doesn't take into account presence of other toxicants or contaminants in the water).
Moreover, it is important to emphasize that assessment of the toxicological relevance of a hazard needs to undertaken based on rigorous scientific evidence. This needs to undertaken within an overall safety assessment framework that considers the relevance of the hazard(s), toxicokinetics of the test substance as well as its dose-response characteristics and identified risk(s).
Based on what has been presented in this section, a key take-away is that the mere presence of a hazard does not imply the presence of a meaningful risk and does not necessarily mean a substance is to be avoided. For example, certain natural substances such as vitamin D can be hazardous at high doses (in the case of vitamin D, overdoses can result in calcification of the kidneys, hence its use as a rodenticide (rat poison)). However, in appropriate doses, vitamins like vitamin D are beneficial (and indeed are essential) for optimal health. On the other hand, certain highly toxic compounds such as the alkylating agent cyclophosphamide (a potent genotoxicant) can be life-saving when administered in [small] therapeutic doses as a chemotherapeutic agent for treating certain cancers.
3.3 Toxicokinetics
Toxicokinetics (TK) encompasses the study of factors that determine the time-course kinetics of toxicants in the body. Broadly speaking, this involves assessment of the: 1) Absorption; 2) Distribution; 3) Metabolism; and 4) Excretion / elimination (collectively termed "ADME") of compounds within the organism. Toxicodynamics (TD) assesses the effects of substances in a biological system or tissue. Assessment of the TK and TD properties of a chemical are necessary to relate internal exposure of a test material (or its active metabolite(s)) at its target site(s) of action to the administered doses of the test substance.
3.3.1 Absorption
For most chemicals, the principal routes of exposure are via the: 1) Oral (ingestion); 2) Inhalation; and 3) Dermal modes. Additional exposure routes include intravenous (IV), intraperitoneal (IP), subcutaneous and intramuscular (IM) but these are primarily seen in relation to drug administration or in vivo dosing scenarios. Absorption of chemicals via the gastrointestinal (GI) tract and the lungs represent the most efficacious method of administering test substances. It is important to note that IV dosing adminsters the test substance directly into the bloodstream, thereby bypassing the need for it to be absorbed. The stratum corneum of the skin renders it a less favorable route for absorption of xenobiotics (for the most part, exceptions include skin sensitizers such as urishiol and transdermal medications which are specifically formulated to be absorbed via the skin).
3.3.2 Distribution
Once absorbed, xenobiotics are distributed via the blood and taken up into target organs and tissues. Both passive and active transport mechanisms are involved in distributing compounds to their sites of action. Certain compartments, such as the central nervous system, have a semipermeable barrier to selectively regulate xenobiotic transport into the compartment (in the case of the CNS, this is termed the "blood-brain barrier"). These barriers are not perfect and can fail. An example is with collie-type dogs who have a high prevalence of a mutation in p-glycoprotein (a multi-drug transporter called MDR1) which renders it non-functional and as a result, the blood-brain barrier less effective at excluding ivermectin and related anti-parasitic drugs. As a result, these breeds are uniquely susceptible to the neurotoxic effects of these drugs.
3.3.3 Metabolism
Box 1: Some Major Phase I and Phase II Biotransformation Reactions
- Phase I Reactions
- Oxidation
- Reduction
- Hydrolysis
- Dehalogenation
Phase II Reactions - Glutathione (GSH) conjugation
- Glucuronidation
- Sulphation
- Acetylation
- Amino acid conjugation
Metabolism (also called biotransformation) refers to a broad set of enzymatic reactions undertaken by the body to alter the structure of xenobiotics. Broadly speaking, metabolism involves converting lipophilic xenobiotics (which are readily absorbed) into more hydrophilic forms for excetion (e.g., via the urine).
There are 2 main types of metabolic reactions: 1) Phase I reactions refer to a family of oxidation (primarily catalyzed by the cytochrome p450 family of enzymes), reduction and hydrolysis reactions which serve to make the xenobiotic more polar; and 2) Phase II reactions which involve conjugation of the xenobiotic to make it more hydrophilic and allow for its clearance. In certain cases (as with the previous discussion on TOCP), phase I biotransformation of the parent molecule can serve as an activating step, metabolizing the chemical into its active (toxic) form. A key point to remember is that biotransformation reactions are catalyzed by various enzymes that fall into the broad classes highlighted in Box 1. These include oxidases (e.g., the cytochrome P450 family of enzymes), hydrolases (e.g., carboxylesterase, paraoxonases), reductases (e.g. alcohol dehydrogenase), etc.
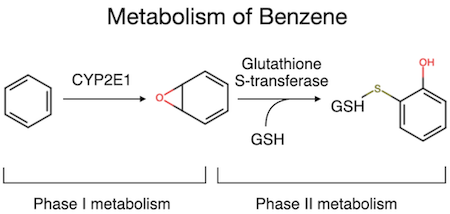
An example of phase I and II metabolism involving benzene is shown in Figure 3. In this case, Cytochrome P4502E1 (CYP2E1) catalyses the phase I biotransformation of benzene to form benzene oxide, which is chemically reactive. Benzene oxide then undergoes phase II metabolism via conjugated to glutathione. In reality, biotransformation pathways can be complex, with major and minor paths being present. Also, when undertaking an in vivo or in vitro metabolism study, it is important to consider species differences to ensure that all metabolites expected to be present in the human are also present in the test system at similar levels (i.e., there are no human-unique or over-represented metabolites).
3.3.4 Excretion
Excretion (or elimination) refers to the process by which the body eliminates substances. This is primarily via the urine, exhaled air and bile. Excretion via the urine is the most important route of elimination, although other modes (such as elimination into other bodily secretions such as milk, sweat and tears) are also possible. In general, biotransformation of a xenobiotic to a more hydrophilic form is a prerequisite to urinary excretion, which favors excetion of smaller xenobiotics (typically those with molecular weights < 350 Da).
Acknowledgements
- Dr. Rudy Richardson, Dow emeritus professor of toxicology at the University of Michigan.
External resources
- ToxTutor: A free online toxicology resource originally developed by the National Library of Medicine (NLM)
- Casarett & Doull's Toxicology: The go-to text book for toxicologists
- Patty's Toxicology: A major reference for toxicological and industrial hygiene information on a vast array of chemicals
References
- 1 Cartoons have been adapted from AI-generated images from Gemini 2.0 Flash or Gemini 2.5 Pro; Google, April 2025, https://gemini.google.com.
- 2 Taken from ChemID+ data for water (CAS: 7732-18-5) in PubChem.
- 3 Taken from ChemID+ data for potassium cyanide (CAS: 151-50-8) in PubChem.
- 4 Taken from ChemID+ data for ethanol (CAS: 64-17-5) in PubChem.